Shivayogi Hiremath1,2, MS, Dan Ding1,2, PhD , Christopher Okonkwo1,3, Matthew Hannan1,4, Rory Cooper1,2, PhD
1Human Engineering Research Laboratories, Department of Veterans Affairs, Pittsburgh, PA
2Department of Rehabilitation Science and Technology, University of Pittsburgh, Pittsburgh, PA
3Department of Computer Science, Norfolk State University, Norfolk, VA
4College of General Studies, University of Pittsburgh, Pittsburgh, PA
ABSTRACT
Regular physical activity (PA) is crucial in wheelchair users and is associated with a number of health benefits. One way of assisting wheelchair users to attain the recommended PA level is to provide them with tools that help quantify their PA by providing near-real-time feedback. The objective of this study was to test the performance of a gyroscope based wheel rotation monitor (G-WRM) that can estimate speeds and distances travelled by manual wheelchair users (MWUs) during wheelchair propulsion and handcycling. The results of this study indicate that G-WRMs can accurately (>95%) measure angular velocities and distances travelled by MWUs during wheelchair propulsion and handcycling. In addition, excellent ICC values and Bland and Altman plot agreement indicate that the G-WRMs are reliable and valid compared to various criterion measures such as measured distances, a SmartWheel and a motion capture system. The G-WRMs can be used to monitor mobility characteristics in MWUs.
INTRODUCTION
Regular optimal physical activity (PA) is crucial in wheelchair users as it is associated with a number of benefits such as increased aerobic capacity, muscular strength and endurance, flexibility, improved psychological well-being, and reduced risk of cardiovascular disease and other chronic conditions (Glaser, Janssen, Suryaprasad, Gupta, & Mathews, 1996). However, the environmental barriers and lack of accessible equipment in addition to the physiological changes in wheelchair user population has resulted in low levels of regular physical activity (Hoenig, Landerman, Shipp, & George, 2003; U.S. Department of Health and Human Services, 2011). Research has shown that it is possible for wheelchair users to attain energy expenditure levels that are comparable to the PA recommendations of the American College of Sports Medicine (ACSM) for general population by participating in wheelchair basketball, wheelchair tennis or handcycling (Abel, Platen, Vega, Schneider, & Struder, 2008; Valent et al., 2008). One way of assisting consumers to attain the recommended PA level is to provide them with tools that help them quantify their PA by providing near-real-time feedback.
Literature review has indicated that there are various types of monitoring devices used by researchers to capture mobility characteristics of wheelchair users. Tolerico et al.’s group evaluated a pendulum and reed switch based wheel rotation datalogger to capture mobility characteristics of manual wheelchair users (MWUs) (Tolerico et al., 2007). The study found that MWUs used their wheelchairs for a mean (SD) distance of 6,745.3 (1,937.9) m at a speed of 0.96 (0.17) m/s and 2,457.0 (1,195.7) m at a speed of 0.79 (0.19) m/s in the National Veterans Wheelchair Games and community, respectively (Tolerico et al., 2007). Researchers have also investigated the use of a wheel mounted tri-axial accelerometer to detect wheel revolutions and distance travelled (Coulter, Dall, Rochester, Hasler, & Granat, 2011; Sonenblum, Sprigle, Caspall, & Lopez, 2012). Coulter et al. found that the wheel mounted accelerometer was accurate in estimating wheel revolutions, absolute angle and duration of movement (ICC > 0.999, 0.999, 0.981, respectively) in 14 MWUs who propelled along a course (Coulter et al., 2011). Sonenblum et al. found that the wheel mounted accelerometer had an accuracy greater than 90% for various wheelchair and wheel types, propulsion techniques, speeds, and wheelchair-related activities of daily living in two MWUs and two individuals without disability (Sonenblum et al., 2012). Our literature review indicates that none of these monitors have been tested for estimating speeds and distances from regular wheelchair propulsion to wheelchair sports such as handcycling. In addition, these monitors do not provide real-time feedback about mobility characteristics to wheelchair users.
The objective of this study was to test the performance of a gyroscope based wheel rotation monitor (G-WRM) that can estimate speeds and distances travelled by manual wheelchair users. We evaluated the validity of the G-WRM in estimating speeds and distances through a series of laboratory-based tests including bench tests and simulated wheelchair use tests. The wheelchair use tests included wheelchair propulsion as well as handcycling.
METHODOLOGY
The choice of using a gyroscope sensor based wheel rotation monitor was based on a pilot study and laboratory tests that indicated that the accelerometer and reed switch based dataloggers were not suitable for speeds such as handcycling. The accelerometer signals in the plane of wheel rotation separated and saturated for speeds higher than regular propulsion such as handcycling. Similarly, the reed switch and pendulum based datalogger underestimated speeds whenever a wheelchair traveled with speeds greater than 2.5m/s (5.6miles per hour).
Instrument
The G-WRM shown in Figure 1 is a rechargeable device that can be attached to the spokes of the wheel of a wheelchair or a handcycle. The G-WRM contains six reed switches that are triggered by a pendulum and magnet assembly and a two-axis gyroscope sensor which allows us to capture speeds varying from wheelchair propulsion to handcycling. However, in this study we have only used the G-WRM’s gyroscope to measure angular velocities, which were then converted into speeds and distances traveled. Additionally, the G-WRM contains a Bluetooth module that can be used to send data about the user’s mobility to a smartphone, and a micro secure digital memory card that can be used to store the data on the device locally.
Calibration
The calibration of the G-WRM involved collecting raw gyroscope data from the device while it ran at fixed angular velocities on a ST20 Computer Numerically Controlled (CNC) lathe (HAAS Automation, Inc., Oxnard, CA). The gyroscope data was collected from repeated trials of two minute durations at speeds of 40 rotations per minute (rpm), 60 rpm and 80 rpm, respectively, in both clockwise (forward) and counterclockwise (reverse) directions. The gyroscope data was then used to develop offset values and basic calibration equations for each of the G-WRM in both forward and reverse directions to estimate various angular velocities. The angular velocity information along with the wheel diameter was then used to estimate linear speeds and distances travelled by wheelchair users.
Protocol
We evaluated the reliability and validity of three G-WRM prototypes in measuring angular velocities, and estimating speeds and distances travelled by a wheelchair and handcycle through laboratory and wheelchair use tests. The wheelchair use tests were performed by a manual wheelchair user with spinal cord injury and an experienced wheelchair user without a disability.
We performed CNC lathe tests to evaluate the reliability and validity of the G-WRM in measuring the angular velocities. The G-WRMs were secured to a lathe and ran for ten minutes of duration each at angular velocities of 40 rpm, 60rpm, and 80rpm in both forward and reverse directions for three times. Along similar lines we evaluated the reliability and validity of the G-WRM in estimating linear speeds by performing double drum tests. The G-WRMs were secured to the spokes of a manual wheelchair set up on a double drum (ISO 7176-08) with drive wheels on one drum and castors on the other drum. The front and back double drums ran at 0.95m/s (2.12 miles per hour) and 1m/s (2.24 miles per hour), respectively, to simulate road hazards commonly encountered by wheelchair users in natural environments. The tests were repeated twice for a period of 6 hours in both forward and reverse directions.
Sl. No. | Propulsion Task |
---|---|
1. |
Straight forwards (10m) on a flat tile surface |
2. |
Straight forwards (15m) on a flat tile surface |
3. |
Straight forwards (20m) on a flat tile surface |
4. |
Straight backwards (10m) on a flat tile surface |
5. |
Up and down a ramp (slope of 2.7°, length 12.19m) on a flat tile surface |
6 |
Straight forwards (18m) on a flat tile surface |
The validity of the G-WRM in estimating distances travelled during wheelchair propulsion was evaluated by performing 6 tasks (Table 1). Each of these tasks was repeated 6 times. An investigator experienced in wheelchair use propelled a manual wheelchair with a camber of 2.5°. The criterion measure for the first 30 trials was a tape measure for fixed distances. The G-WRMs were secured to the spokes of the manual wheelchair’s wheel while the investigator performed the test. The criterion measure for the distances travelled during the last 6 trials were a SmartWheel (Three River Holdings, Inc. Mesa, AZ) and a 3-D passive motion capture system (model MX, Vicon Peak; Lake Forest, California). The G-WRM devices were secured to the spokes of the SmartWheel. The VICON and SmartWheel were calibrated as per the manufacturer's specifications.
Furthermore, the validity of the G-WRM in estimating distances travelled during handcycling was evaluated by attaching the G-WRM to the spokes of an Invacare Top End Force R X handcycle (Invacare Corporation, Elyria, OH). The handcycling test was performed by an investigator who is an experienced wheelchair user with disability. The test involved handcycling for nine laps on a cycling track. The criterion measure for the distance travelled was the total track length of 7.24km (0.804 km for 9 laps).
Data Collection
The data collected from the G-WRM included gyroscope sensor data at a sampling rate of 64Hz (15.62 millisecond). An Android based smartphone paired with G-WRM via Bluetooth was used to wirelessly collect data from the G-WRM for all trials. We used the angular velocities detected by the G-WRM’s gyroscope sensor along with the calibration equations to calculate the speeds and distances travelled.
Statistical Analysis
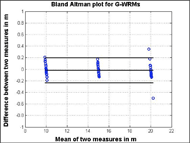
Figure 2: Bland Altman plot of distances measured using tape measure versus distances estimated from the G-WRMs during wheelchair propulsion trials for 10m, 15m and 20 m distances.
The data analysis software to process and analyze data from the G-WRM and criterion measures was written in MATLAB® (Version 7.12 R2012b, The Mathworks Inc. 223 MA, USA). The comparisons between the G-WRM variables estimated by G-WRM (angular velocities and speeds and distances travelled) and criterion measures were performed by calculating the absolute difference, mean difference, and percentage errors for each trial. Additionally, Intraclass correlation coefficients for single measure using two-way mixed model with consistency (ICC (3,1)) and Bland and Altman plots were used to assess the agreement between the estimated measures from the G-WRM and the criterion measures. All statistical analysis was performed using IBM SPSS software (ver. 20.0, IBM Corporation NY, USA), with the statistical significance at an alpha level of 0.05.
RESULTS
Tests | Absolute Error in percentage (%) | Mean Percentage Error (SD) |
---|---|---|
CNC Lathe |
||
Forward and Backward at 40 rpm |
0.26 |
0.04 (0.39) |
Forward and Backward at 60 rpm |
0.32 |
0.06 (0.34) |
Forward and Backward at 80 rpm |
0.35 |
0.09 (0.38) |
Double Drum |
||
Forward and Backward |
0.89 |
-0.37 (0.95) |
Figure 3: Bland Altman plot of distances estimated using VICON versus distances estimated from the G-WRMs during wheelchair propulsion trials for a distance of 18m.
Tables 2 shows the results from the CNC lathe and double drum tests. Table 3 shows the results from the wheelchair propulsion tests. The ICC(3,1) values for the three G-WRMs for the forward propulsion trails (10m,15m, and 20m) varied from 0.999 – 1.000 (Lower bound: 0.999 – 1.000 and upper bound: 1.000 –1.000). Figures 2 and 3 show the Bland and Altman plots used to assess the agreements between the G-WRMs and the criterion measures during the wheelchair propulsion tests. The percentage errors for distances estimated by the G-WRMs for the handcycling test varied from -0.88% to 1.06%.
Propulsion Test |
Absolute Error in percentage (%) |
Mean Percentage Error (SD) |
Forward (10m) |
0.56 |
-0.30 (0.71) |
Forward (15m) |
0.70 |
-0.10 (0.93) |
Forward (20m) |
0.47 |
-0.10 (0.57) |
Backward (10m) |
0.59 |
-0.33 (0.63) |
Forward on a ramp |
0.60 |
-0.43 (0.58) |
Forward with SmartWheel (18m) |
0.50 |
0.07 (0.58) |
Forward with VICON (18m) |
0.59 |
-0.38 (0.96) |
DISCUSSION
Monitoring and providing feedback about mobility characteristics in wheelchair users can help empower them to lead a healthy and active lifestyle. In this study we have shown that G-WRMs can accurately capture speeds and distances traveled by MWUs for speeds varying from wheelchair propulsion to handcycling and can provide a near-real-time feedback through an Android based smartphone.
The results of this study indicate that G-WRMs can accurately (>95%) measure angular velocities and distances travelled by MWUs during wheelchair propulsion and handcycling. In addition, excellent ICC values and Bland and Altman plot agreement indicate that the G-WRMs are reliable and valid compared to various criterion measures. Comparing the results of this study with the research of Coulter et al. and Sonenblum et al. we find that the G-WRMs have similar performance making it suitable for collecting daily mobility parameters in MWUs (Coulter et al., 2011; Sonenblum et al., 2012). However, the advantage of using a gyroscope sensor over an accelerometer is that we can directly obtain the angular velocity information reducing the computational need and can collect mobility characteristics for sport related activities such as handcycling. Furthermore, the ability to use G-WRMs with Smartphones will allow clinicians and researchers to perform longitudinal studies of wheelchair use in community settings.
Limitations of this study include testing the G-WRMs in only two participants and the inability of the device to detect self-propulsion versus external pushing. In future, we plan to test the device in 45 MWUs and also try to combine G-WRMs with body worn sensors to detect self-propulsion versus external pushing.
CONCLUSIONS
To our knowledge this is the first study to validate a gyroscope based wheel rotation monitor to estimate speeds and distances travelled by wheelchair users. The G-WRMs can be used to monitor mobility characteristics in MWUs.
REFERENCES
U.S. Department of Health and Human Services, Washington, DC. (2011, Jan 10, 2011). Healthy People 2020., from http://www.healthypeople.gov/2020/topicsobjectives2020/overview.aspx?topicid=9
ACKNOWLEDGEMENTS
The work is supported by the Department of Defense (W81XWH-10-1-0816) and the National Science Foundation’s QOLT REU (EEC 1063017) and ELeVATE (EEC 1036964) programs. Mr. Hiremath's work on this article was funded through the Switzer Research Fellowship (H133Fl10032) awarded by the National Institute on Disability and Rehabilitation Research, Department of Education. The work is also supported by the Human Engineering Research Laboratories, VA Pittsburgh Healthcare System. The contents do not represent the views of the Department of Veterans Affairs or the United States Government.